Shedding Light on Human Cerebral Cortical Folding and Channelopathies
"The RNAscope assay was very helpful in discovering this, not only because we were able to confidently discern between the different sodium channels, but also because we could use the RNAscope Multiplex Fluorescent kit v2 in situ assay to co-localize gene expression with cell types."
- Richard S. Smith, PhD, Post-Doctoral Research Fellow in the Laboratory of Christopher A. Walsh at Boston Children’s Hospital, Harvard Medical School, Howard Hughes Medical Institute
Channelopathies are responsible for a range of brain disorders and are caused by abnormal ion channel function. There is a need to better understand the underlying pathophysiology of these channel-based disorders, as individuals presenting with clinical phenotypes are difficult to diagnose and treat. Post-Doctoral Research Fellow at Harvard Medical School, Dr Richard S. Smith, spoke to us about how his research in this area has shed light on an unexpected association between one particular channelopathy, connecting sodium channels to cortical folding and brain development. He explains how the RNAscope ISH assay, with its specificity and multiplex capabilities proved a critical assay for discovering novel findings that provide an important diagnostic marker, and challenge the accepted dogma of human cerebral cortical folding.
Can you tell us about your background and work?
I’m a neurobiologist, having completed my PhD with Dr Ricardo Araneda at the University of Maryland. My work there involved patch-clamping individual single neurons and looking at network processes. Since then, my research has developed from an electrophysiological to a molecular and a genetics perspective, in the Laboratory of Dr Christopher Walsh. At the Walsh lab we study childhood developmental disorders. Genetic screening gives us the ability to uncover new neurodevelopmental information and insights into the biology of these diseases. Many individuals that we enroll in our research program have cortical malformation diseases or developmental disabilities, and families have no answers for what is the basis of their diseases. So, it’s really wonderful whenever we solve a case, not only because we are elaborating on the genes involved in diseases, but also because we’re directly helping families. We receive emails from people all around the world once we publish a new gene related to a disorder. Getting positive feedback on the work we’re doing in helping people is a great source of daily inspiration.
Your recent paper published in Neuron1 has had a major impact in the field of channelopathies. Can you tell us more about this work?
In this study, we evaluated the role of sodium channels in the developing human brain. It’s classically thought that sodium channels in the brain or nervous system are primarily involved in the transmission of electrical signals via action potentials. However, one of the surprising things that we discovered is that it seems these sodium channels are involved in shaping the growth and the blueprint of the embryonic circuit for the human cortex, from weeks 14 to 25 of embryonic gestation, far before action potentials reliably occur.1 There appears to be a very important period in fetal development that relies on sodium channels to promote the formation of the cerebral cortex in a way that we didn’t expect; not necessarily using action potentials, but perhaps another type of slower sodium flux mediated by these channels, to affect the formation of gyri and sulci in the cortex (Figure 1).
Channelopathies are often thought of as diseases of post-mitotic circuits – fully-formed circuits that don’t function properly, for example epilepsy. However, in the disease that we studied - which we’re calling a developmental channelopathy - the pathophysiology is rooted in the circuit that doesn’t form properly. It appears that during development, neurons don’t follow the correct type of growth patterns and so they don’t form the final circuit as in healthy tissue. However, once the circuit is fully formed, the electrical dysregulation doesn’t appear to grossly affect output of the neural circuit, which means that we don’t observe epilepsy in many of these adult individuals.
What we have found is that the region of the brain, which the disease seems to be affecting, has important centers for language and speech production abilities. As such, many of the patients in our study have altered or dysarthric (slurred or slow) speech properties and/or oral motor development issues, and we can correlate this. The particular type of brain disease that we studied is called “perisylvian polymicrogyria”, which comes from Greek meaning many-(poly) small-(micro) brain folds. Affected individuals have brains that look similar in surface texture to a cauliflower along the sides of the cortex (Figure 2). Studying the brains of familial groups of individuals enabled the identification of the gene underlying this disease, SCN3A, which codes for a specific type of sodium channel, NaV1.3.
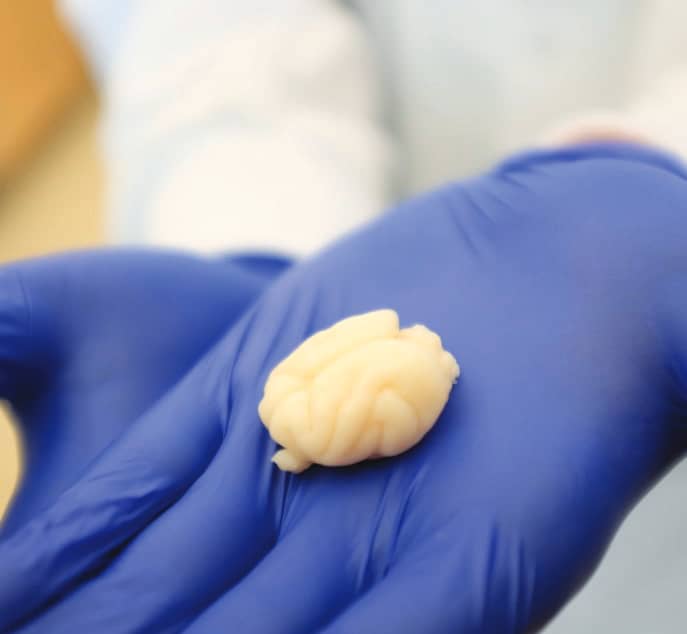
Figure 1. Ferret brains possess gyri and sulci (peaks and valleys), similar to humans, and were used for in vivo modelling in the Smith et al., study.1
How did you discover the SCNA3 gene in relation to this channelopathy?
This study originated from one of our collaborators, Maria Lehtinen. She was working with a large multi-generational family in Finland for many years from the laboratory of Anna-Elina Lehesjoki, before she started her own lab after a postdoc in the Walsh Lab at Boston Children’s Hospital. The family is the largest polymicrogyria family described to date - four generations with three affected sibling groups, which is very large for a genetic tree and gave us really good clinical phenotyping. We had great clinical collaborators involved with this family for 20 plus years, so there is very strong historical data on these individuals and their pathologies over time. This particular polymicrogyria was a heterozygous disease - only one allele in the SCN3A gene is affected in individuals with clinical phenotypes. One of the things that made identifying the underlying genetic cause difficult is that mutations in channel genes resulting in channelopathies, have decreased penetrance. This means is that an individual can carry the disease allele without having the disease phenotype, but can pass this allele to children who can have the disease. This decreased penetrance of SCN3A mutations slowed the study for many years when we were trying to figure out exactly which allele was responsible. However, once we isolated it in one family, found some more, it became very obvious that the SCN3A gene and the associated NaV1.3 sodium channel, when mutated, was able to produce these profound neurological deficits.
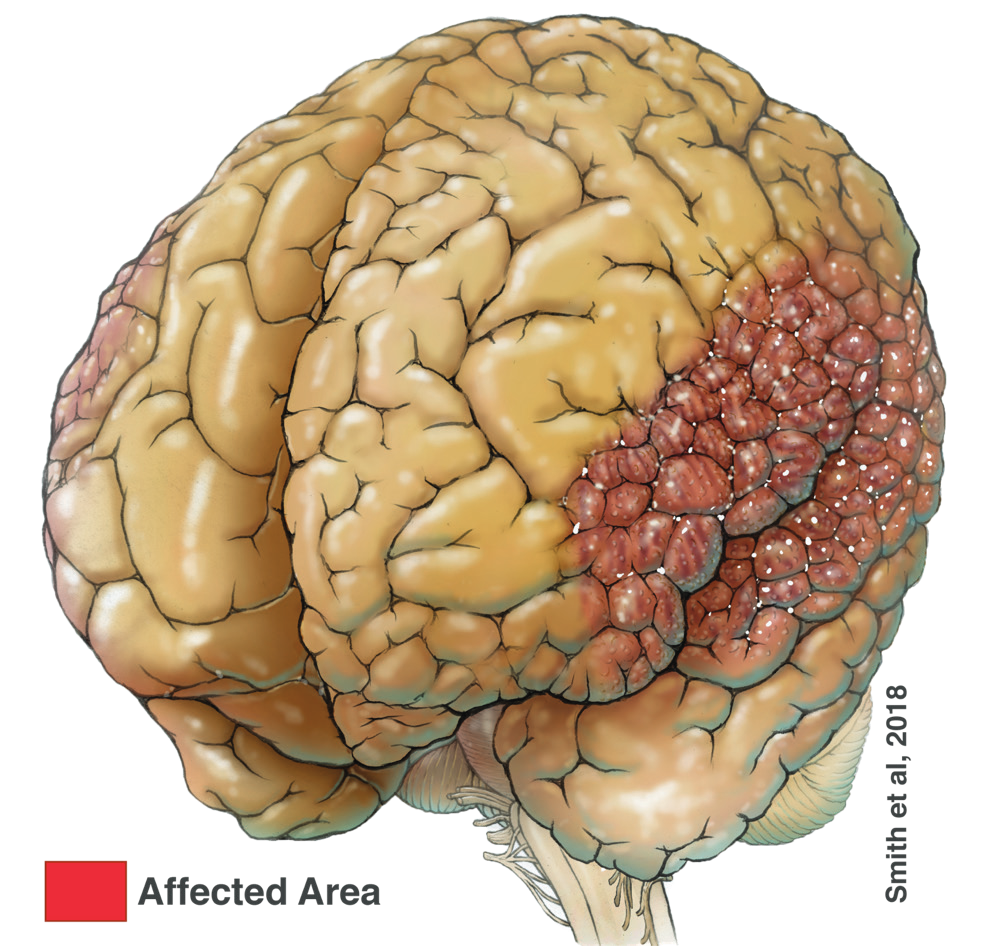
Figure 2. Illustration of Perisylvian Polymicrogyria (red area).
Once you identified SCNA3, where did you go from there?
This for me, is the very exciting part. We looked at the particular sodium channel and where it’s expressed. This is where I began my interactions with ACD and using the RNAscope in situ hybridization technology. One of the difficulties of working with sodium channels and other transmembrane receptors, is that these channels are duplicated many times in our genomes, and therefore have a great deal of similarity in their genetic codes. There are multiple copies of the sodium channel gene and approximately 10 sodium channel types. The body uses these channels in different places and at different times. In the brain, there are four channel types that are remarkably similar in amino acid structure and properties. It’s very difficult then to use classical methodologies, such as antibodies, and be 100% sure that you’re detecting the specific type of sodium channel that you want to study. In our case, we wanted to study SCN3A and we had to be sure that we weren’t seeing any transcripts from SCN1A or SCN2A, particularly as we knew from the single cell data that SCN2A is present in some embryonic cortical cells.
The really important advantage of using the RNAscope technology was that we were able to develop probes to the specific sequence differences between these channels. When looking at SCN3A versus SCN2A for example, we could be very confident in our findings that a particular gene is expressed in a particular cell type in the human fetal cortex at a particular time point. That was vital to lay the basis of how a sodium channel could create and develop into diseases in these patients.
How did the RNAscope assay contribute to your findings?
Before this work, polymicrogyria was largely thought to have mutations in genes involved in structural properties, such as microtubules and cell division. This study has uncovered a new paradigm, in terms of a new mechanism of action that can affect the development of the growing cerebral cortex.
The RNAscope assay was very helpful in discovering this, not only because we were able to confidently discern between the different sodium channels, but also because we could use the RNAscope Multiplex Fluorescent kit v2 in situ assay to co-localize gene expression with cell types. For example, for radial glial cells we used specific markers, such as TBR2 or other indicators of this population and then looked to see if they were co-localized with our sodium channel of interest.
In this way we not only detected the cell types, but also detected them in relation to gene expression in a very specific and precise way. This enabled us to say with certainty that this is a specific population of cells, which we know are a dividing population of cells – something we were very skeptical about when we began to hypothesize the underlying cause of the disease phenotype (Figure 3).
Figure 3. Pathophysiology of perisylvian polymicrogyria and SCN3A in situ gene expression pattern, identified with ACD’s highly specific RNAscope assay. (CP – cortical plate, SP – subplate zone, IMZ – intermediate zone, SVZ – subventricular zone, VZ - ventricular zone).
Why was the SCNA3 expression pattern during development important?
One of the interesting things that we’ve learned from this study was that SCN3A seems to be enriched in embryonic development and tapers off postnatally. Which means that there are higher amounts of this protein during early fetal development when action potentials are sparse, and after birth it tapers off by several years of age, before the teens.
This finding is important because it is in stark contrast to the accepted understanding of sodium channels in relation to NaV1.3. We normally think of NaV1.3 as being involved in action potential upstroke. This is an interesting finding as its noncanonical – the channel is functioning in dividing cells in the cerebral cortex as well as in cells in the neuronal layer
When we patch-clamped these cells to evaluate their electrophysiological properties, we found that they didn’t produce action potentials, which lends support to the hypothesis that the sodium fluxes' primary role is not to create action potentials in the embryonic circuit. Instead we believe that the sodium flux is important for other downstream effects, for example, sodium and calcium exchangers ATPase rely on having a sodium gradient. There are several other effector functions of sodium, such as activating calcium channels. Sodium could be doing several functions across cells that isn’t action potential generation.
This finding is important because it defines a new category of this disease. Patients who present with polymicrogyria will be genetically screened for mutations in SCN3A. In this regard, it’s a very helpful discovery, but it’s also really important because we now have an idea that this particular gene is involved in the development of the cerebral cortex. There’s a lot of important biology underlying the diagnosis of patients that we’re continuing to explore.
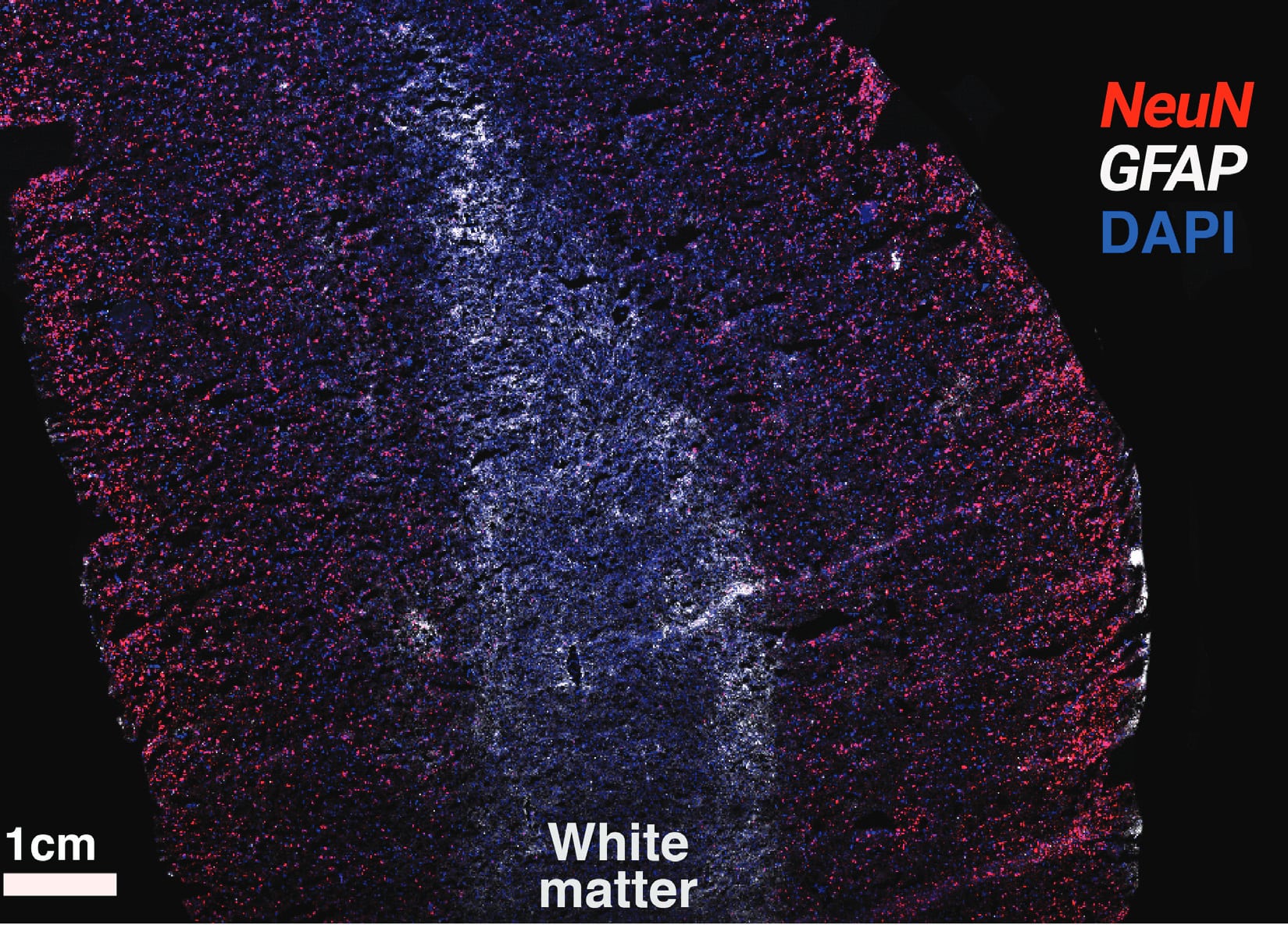
Figure 4. Detection of NeuN (red) and GFAP (white) in human brain tissue with the RNAscope Multiplex Fluorescent kit v2. Cells are counterstained with DAPI.
How was your experience of working with ACD?
ACD has a great probe development team that we worked with - all you need to do is specify the particular genes you want to look at and they do the rest. For this study, we selected the three genes and said that we wanted to make sure that there wasn’t any crosstalk between them – and they were very happy to help.
In the past, we’ve also worked with ACD’s teams to develop other probes where we’ve specified where exactly we wanted to design the probes against. That’s mostly been for isoform-specific research, for example, where we know we have two different, alternatively expressed exons in the same piece of tissue.
In addition, the ACD’s support team was very helpful with our tissue. We used the RNAscope Multiplex Fluorescent Assay v2 with some protocol modifications as our tissue was very delicate. The tissue doesn’t have much extracellular matrix or supporting cells, so it doesn’t hold together very well. The support team was very helpful in giving us tips about ways to better handle the tissue and we formed a modified tissue prep protocol. The V2 kit worked very well for confidently detecting the transcripts and analyzing them in the human fetal cortex.
What will you be working on next?
There’s a lot of interesting mechanisms underlying how SCN3A is involved in this polymicrogyria, so we’re looking at downstream targets and possible genetic interventions. For example, trying to target and rescue this gene in animal models and looking at ways to modulate the channel to better understand how sodium effects cells in the developing cerebral cortex. We have also been trying to discern and evaluate the role of other sodium currents in these individuals.
In addition, we know from the literature on epilepsy drugs that there is a significant rate of major malformation in the cerebral cortex in children of women who are treated with anti-epileptic drugs when pregnant—which often target sodium channels. This effect depends on the particular sodium channel modulator or the anti-epileptic drug. We’re investigating ways to improve treatment for these women.
As a whole, we want to better understand the role of sodium in the growth of the human fetal cortex. There’s a lot of interesting avenues moving forward, both from a clinical perspective - for polymicrogyria patients and pregnant women who are on anti-epileptic modulators, and also from a basic biology perspective - understanding why this mutated channel is causing brains to over-fold.
Selected Publications
-
Smith, R.S. et al. (2018) Sodium Channel SCNA3 (NaV1.3) Regulation of Human Cerebral Cortical Folding and Oral Motor Development Neuron 99:905.